Syndecans in Elastogenesis and Wound Healing
About the Author: Ms. Ramshaa Mahalingam is an upcoming Master’s Student in the Reinhardt Lab in the Faculty of Dental Sciences and Oral Health at McGill University (Montreal, Canada). Her research study focuses on the mechanisms involved in interaction of cells with extracellular matrix components during elastogenesis. This month in the CCTS “Research and Lab Highlight Series”, Ramshaa shares her take on the current information in the connective tissue field about elastogenesis and also about her exciting new research project.
The elastic fiber system confers elasticity to various vital organ systems and connective tissues. These include tissues such as blood vessels, skin, and lungs, wherein the elastic fibers provide the necessary elasticity and allowing recoiling to enable their proper function [1]. These fibers mainly consist of elastin and fibrillin-1 microfibrils, and the assembly of this fiber system is highly regulated by complex spatial and temporal regulation of multiple factors [2]. The process of elastic fiber formation is termed elastogenesis which starts during early embryogenesis at about mid-gestation. Elastogenesis begins with the synthesis and secretion of the precursor form of elastin, called Tropoelastin (TE), a ~70 kDa protein encoded by the ELN gene and synthesized in the rough endoplasmic reticulum (ER) [1,3,7] (Figure. 1). TE is secreted by elastogenic cells such as smooth muscle cells, fibroblasts, myofibroblasts, and endothelial cells. Upon secretion, tropoelastin undergoes self-aggregation and coacervation on the cell surface which is facilitated by several accessory proteins such as fibulin-4 and -5 (FBLN4/5) and a chaperone called elastin-binding protein (EBP) [4,5,7]. Then, this complex is deposited onto microfibrils that are predominantly made of fibrillin-1 (FBN1) and fibrillin-2 (FBN2), which serve as scaffolds for the assembly of elastic fiber [6, 18]. The long isoform of latent TGFβ-binding protein 4 (LTBP-4L) is subsequently required for the recruitment of these TE microassemblies onto the microfibrils. It was discovered by Kumra et al. that FBLN4 acts as a chaperone to unfold LTBP4L facilitating the deposition of TE onto the elongated LTBP4L [6,16]. As mentioned by Noda et al., FBLN4 is also required for the activity of lysyl oxidase (LOX) [17]. FBLN4 oversees transportation of copper ions from the copper transporter ATP7A to lysyl oxidase (LOX) in the trans-Golgi network in order to facilitate LTQ formation and thereby the activation of LOX [17]. This copper-dependent enzyme is responsible for creating hydroxylysine residues on TE which allows multiple TE proteins to crosslink with one another advancing the formation of mature elastic fibers [4] (Figure. 1). The biosynthesis of elastin gradually decreases with age and de novo synthesis of this protein does not occur during adulthood [1,2,3,4,6]. TE, fibronectin (FN) [19,20], fibrillins (FBN1-2) [19], fibulins (FBLN4-5) [18], and LTBP-4 [16] are important proteins involved in elastogenesis and mutations in any of these proteins are known to cause heritable connective tissue disorders such as Cutis Laxa, Marfan Syndrome, Stiff Skin Syndrome, Macular Degeneration, and others.
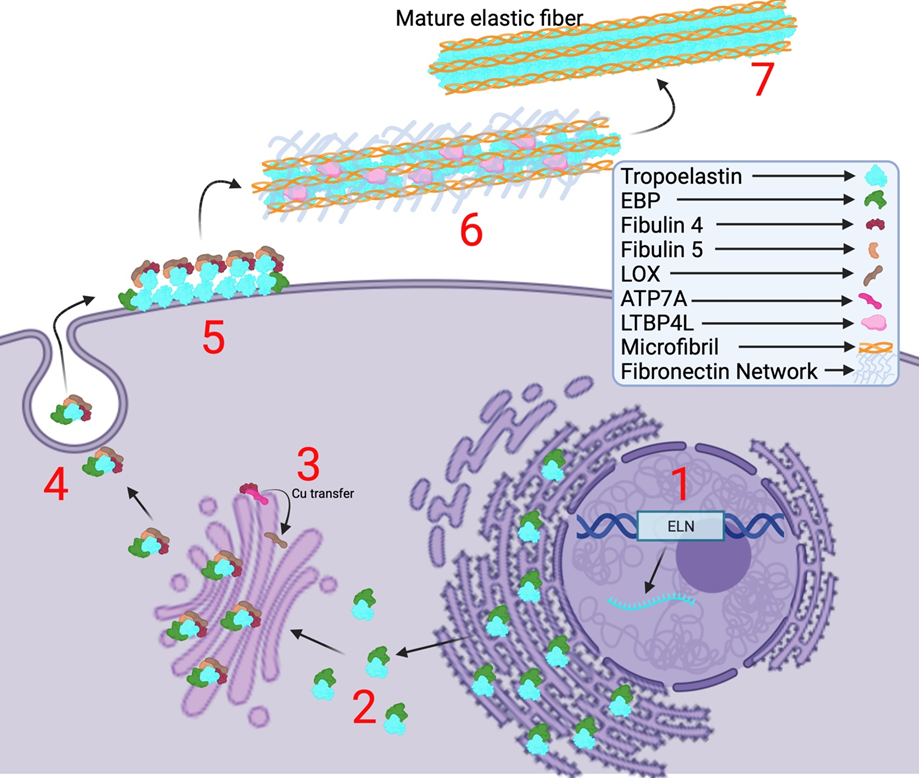
Interestingly, elastogenic proteins FBN1 and FBN2 were also found to play important role during wound healing. For example, Handa et al. investigated the regeneration level of periodontal tissue and the expression of matrix metalloproteinase 9 (MMP9) and tumor necrosis factor-alpha (TNF-α) in FBN1-deficient mice [7]. It was found that reduced levels of FBN1 slowed wound healing compared to wild-type mice, demonstrating that FBN1 is essential for elastogenesis in wound healing. Contrastingly, Brinckmann et al. found that there is an increase of FBN2 expression in wound healing tissues collected from skin biopsies and that they are colocalized with tenascin C [8]. This suggests that elastic fiber proteins are involved in wound healing. However, their extensive role in this process is yet to be discovered.
Wound repair is divided into four continuous stages: hemostasis, inflammation, proliferation, and remodeling [9]. The initial phase consists of the rapid response of preventing blood loss by vasoconstriction and clot formation immediately after vascular damage [9,10]. Vasoconstriction is mediated by prostaglandins, endothelin, catecholamines and prostanoids [9,10]. Additionally, contraction of smooth muscle cells is caused by the platelet-derived growth factor (PDGF) released by platelets that are formed after the subendothelial collagen is exposed to blood [9,10]. Subsequently, these platelets aggregate with one another to form the hemostatic plug which contains other extracellular matrix proteins [9]. During the inflammatory step, leukocytes migrate to the site of injury in order to eliminate microorganisms and cellular debris via the secretion of different chemokines [11]. This phase gradually ends with a decrease in the extravasation and an increase in apoptosis of inflammatory cells [11]. The third proliferative phase consists in repairing the damaged tissue by the proliferation of fibroblast that will differentiate into myofibroblast [12]. This is accompanied by extracellular matrix deposition, more wound contraction, re-epithelialization via the interaction between keratinocytes and fibroblasts, angiogenesis, and peripheral nerve repairs [12]. Finally, the last remodeling step involves strengthening the repaired tissue by matrix metalloproteinases that are secreted by fibroblasts, macrophages, and endothelial cells [13]. Furthermore, all the processes arising after the injury begin to slowly dissipate [13].
With promising advances made in this field, wound healing may be associated with syndecans [14]. Syndecans are a group of four transmembrane heparan sulfate proteoglycans (HSPGs) that all have a short cytoplasmic domain, a transmembrane domain, and a longer extracellular domain [15]. The function of each syndecan is highly dependent on the sequence present in the variable (V) region that lies between the C1 and C2 regions of the cytoplasmic domain [15]. Syndecans are important for fibroblast and endothelial cells proliferation, cell motility, angiogenesis, and the organization of the extracellular matrix [14]. Hence, the central objectives of my upcoming MSc project in the Reinhardt lab at McGill University is to investigate how syndecans interact with elastogenic proteins such as TE, FBLN4/FBLN5, FBN1, FBN2, and LTBPs, and how this affects elastogenesis. Understanding these fundamental cellular interactions and functions will provide us with possible ways to design strategies to enhance tissue regeneration and wound healing.
References
- Zhang, X., Alanazi, Y. F., Jowitt, T. A., Roseman, A. M., and Baldock, C. (2022). Elastic fibre proteins in elastogenesis and wound healing. International Journal of Molecular Sciences 23, 4087.
- Wagenseil, J. E., and Mecham, R. P. (2007). New insights into Elastic Fiber Assembly. Birth Defects Research Part C: Embryo Today: Reviews 81, 229–240.
- Yanagisawa, H., and Davis, E. C. (2010). Unraveling the mechanism of Elastic Fiber Assembly: The roles of short Fibulins. The International Journal of Biochemistry & Cell Biology 42, 1084–1093.
- Halsey, G., Sinha, D., Dhital, S., Wang, X., and Vyavahare, N. (2023). Role of elastic fiber degradation in disease pathogenesis. Biochimica et Biophysica Acta (BBA) – Molecular Basis of Disease 1869, 166706.
- Schmelzer, C. E., and Duca, L. (2021). Elastic fibers: Formation, function, and fate during aging and disease. The FEBS Journal 289, 3704–3730.
- Sun, B., Tomita, B., Salinger, A., Tilvawala, R. R., Li, L., Hakami, H., Liu, T., Tsoyi, K., Rosas, I. O., Reinhardt, D. P., et al. (2021). Pad2-mediated citrullination of fibulin-5 promotes elastogenesis. Matrix Biology 102, 70–84.
- Handa, K., Abe, S., Suresh, V. V., Fujieda, Y., Ishikawa, M., Orimoto, A., Kobayashi, Y., Yamada, S., Yamaba, S., Murakami, S., et al. (2018). Fibrillin-1 insufficiency alters periodontal wound healing failure in a mouse model of Marfan syndrome. Archives of Oral Biology 90, 53–60.
- Brinckmann, J., Hunzelmann, N., Kahle, B., Rohwedel, J., Kramer, J., Gibson, M. A., Hubmacher, D., and Reinhardt, D. P. (2010). Enhanced fibrillin-2 expression is a general feature of wound healing and sclerosis: Potential alteration of cell attachment and storage of TGF-β. Laboratory Investigation 90, 739–752.
- Pérez, L. A., Leyton, L., and Valdivia, A. (2022). Thy-1 (CD90), integrins and syndecan 4 are key regulators of skin wound healing. Frontiers in Cell and Developmental Biology 10.
- Rodrigues, M., Kosaric, N., Bonham, C. A., and Gurtner, G. C. (2019). Wound healing: A cellular perspective. Physiological Reviews 99, 665–706.
- Martins-Green, M., Petreaca, M., and Wang, L. (2013). Chemokines and their receptors are key players in the orchestra that regulates wound healing. Advances in Wound Care 2, 327–347.
- Cañedo-Dorantes, L., and Cañedo-Ayala, M. (2019). Skin acute wound healing: A comprehensive review. International Journal of Inflammation 2019, 1–15.
- Gurtner, G. C., Werner, S., Barrandon, Y., and Longaker, M. T. (2008). Wound repair and regeneration. Nature 453, 314–321.
- Fears, C. Y., and Woods, A. (2006). The role of Syndecans in disease and wound healing. Matrix Biology 25, 443–456.
- Okina, E., Manon-Jensen, T., Whiteford, J. R., and Couchman, J. R. (2009). Syndecan proteoglycan contributions to cytoskeletal organization and contractility. Scandinavian Journal of Medicine & Science in Sports 19, 479–489.
- Kumra, H., Nelea, V., Hakami, H., Pagliuzza, A., Djokic, J., Xu, J., Yanagisawa, H., and Reinhardt, D.P. (2019). FIBULIN-4 exerts a dual role in LTBP-4L–mediated matrix assembly and function. Proceedings of the National Academy of Sciences 116, 20428–20437.
- Noda, K., Kitagawa, K., Miki, T., Horiguchi, M., Akama, T.O., Taniguchi, T., Taniguchi, H., Takahashi, K., Ogra, Y., Mecham, R.P., et al. (2020). A matricellular protein fibulin-4 is essential for the activation of Lysyl oxidase. Science Advances 6.
- Papke, C.L., and Yanagisawa, H. (2014). Fibulin-4 and fibulin-5 in elastogenesis and beyond: Insights from mouse and human studies. Matrix Biology 37, 142–149.
- Sabatier, L., Chen, D., Fagotto-Kaufmann, C., Hubmacher, D., McKee, M.D., Annis, D.S., Mosher, D.F., and Reinhardt, D.P. (2009). Fibrillin Assembly requires fibronectin. Molecular Biology of the Cell 20, 846–858.
- Kumra, H., Sabatier, L., Hassan, A., Sakai, T., Mosher, D.F., Brinckmann, J., and Reinhardt, D.P. (2018). Roles of fibronectin isoforms in neonatal vascular development and Matrix integrity. PLOS Biology 16.